Objective
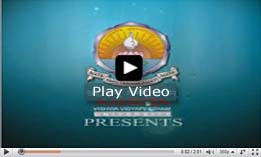
To study the cell migration process.
Theory
Directed cell migration accompanies us from conception to death. This integrated process choreographs the morphogenesis of the embryo during development. The failure of cells to migrate or migration of cells to inappropriate locations can result in life threatening consequences such as the congenital defects prominent in the brain. In the adult, cell migration is central to homeostatic processes such as mounting an effective immune response and the repair of injured tissues.It contributes to pathologies including vascular disease, chronic inflammatory diseases, and tumor formation and metastasis. Understanding cell migration is also becoming important to emerging areas of biotechnology which focus on cellular transplantation and the manufacture of artificial tissues.
Basic Migration Mechanics
Effective cell migration requires the seamless integration of localized, transient signalling events with changes in cellular architecture. Migration is a cyclical process in which a cell extends protrusions at its front and retracts its trailing end. It is spurred into action by migration-promoting or chemotactic agents that induce an initial polarization.
Polarization:
In a quiescent non-migrating cell, adhesive forces maintain the attachment to neighbouring cells and the extracellular matrix. Upon encountering chemotactic molecules, cells re-organize into a predominantly "front", forward moving portion and a predominantly "back", retracting rear portion that are defined by distinct signalling events; this process is known as polarization. Cdc42, PAR proteins (PAR6 and PAR3) and atypical protein kinase (aPKC) are involved in the initial polarization process. Changes in cellular architecture, such as the re-organization of the microtubule-organizing centre (MTOC), microtubules and Golgi apparatus to the front of the nucleus, accompany polarization. The production of phosphatidylinositol trisphosphate (PIP 3) at the leading edge by the action of phosphoinositide 3-kinase (PI3K) is also implicated in polarization. PTEN, a PIP 3 phosphatase, localized to the rear and sides of the cell, helps to regulate the levels of PIP 3. Once polarization is initiated it is maintained by a set of overlapping positive feedback loops involving PI3K, microtubules, Rho family GTPases, integrins and vesicular transport.
Protrusion:
The process of protrusion marks the start of the migration cycle. The actin cytoskeleton provides the basic machinery for protrusion. Actin filaments themselves are intrinsically polarized; fast-growing "barbed" ends and slow-growing "pointed" ends provide an inherent drive for membrane protrusion. This can take the form of spike-like filopodia, in which actin filaments form long parallel bundles, or large, broad lamellipodia, where actin filaments form a branching "dendritic" network. These two forms of protrusion appear to have very distinct functions. The parallel bundle design of filopodia means they are well suited to act as sensors and explore the local environment, whereas the branched design of lamellipodia forms broad protrusions in the direction of migration, providing a strong foundation over which the cell can move forward.
Actin filaments in filopodia elongate at their barbed ends and disassemble releasing actin monomers at their pointed ends, thus, a filament tread-milling mechanism rather than a branched nucleation mechanism is believed to be responsible for filopodial protrusion. The enrichment of proteins such as Ena/VASP,which binds barbed ends and antagonizes capping and branching, and the bundling protein fascin at filopodial tips greatly increases the ability of filopodia to push the plasma membrane forward.
In lamellipodial protrusions a branched nucleation mechanism forms a wide net-like structure which pushes the plasma membrane forward. The Arp2/3 complex induces the formation of new actin filaments by binding to the sides or tip of pre-existing "mother" filaments. The Arp2/3 complex is activated locally at the cell membrane by Wasp/Wave family members which are themselves major targets for Cdc42 and Rac.
The rate and organization of actin polymerization in protrusions is regulated by several actin-binding proteins that affect the pool of available actin monomers and free ends. Profilin binds and targets actin monomers to barbed ends thereby preventing self-nucleation. Capping proteins terminate elongation and limit polymerization to new filaments at the plasma membrane, whereas proteins of the ADF/cofilin family sever filaments and promote actin dissociation from the pointed end. Within the dendritic network of lamellipodial structures other proteins such as cortactin, filamin A and α-actinin help to stabilize branches and cross-link actin filaments.
Traction:
For a cell to advance, newly extended protrusions must attach to the surroundings and stabilize, providing a means of traction for the cell to pull itself forward. The physical component of traction is provided by the action of integrins in adhesions. Tractional force is created at sites of adhesion by the contractile properties of myosin II interacting with actin filaments attached indirectly to integrins at these adhesion sites.
Integrins are a major family of migration-promoting receptors that enable the outer surface of a protruding cell to adhere to the surface over which it is moving. By linking via adaptor proteins to actin filaments, integrins facilitate the cell to pull the bulk of its body forward. Integrins are heterodimeric receptors (comprised of α and β chains) with a short domain projecting into the cytoplasm and a large extracellular domain. They act as both traction sites and mechanosensors transmitting information about the physical state of the surface over which the cell is moving and enabling it to make the necessary cytoskeletal adjustments. Ligand binding induces conformational changes that are transmitted to the cytoplasmic domains, integrin clustering and the activation of intracellular signalling cascades that lead to changes in the rate of phosphoinositol lipid synthesis, protein phosphorylation and activation of small GTPases. These signalling pathways are involved in establishing and maintaining cell polarity, regulating the formation and strength of adhesions as well as in modulating the organization and dynamics of the cytoskeleton. Activated integrins localize to the leading edge of migrating cells and are associated with the formation of new adhesions. While PKC or the GTPase, Rap1 act through talin to promote integrin activation and increase integrin affinity, leading to adhesion assembly, the kinase Raf-1 suppresses integrin activation and favours disassembly.
The turnover of adhesions is critical for effective migration. To extend a protrusion, the adhesions in that portion of the cell must disassemble. Once the protrusion has been extended, adhesions are re-assembled to provide the required traction for the cell to pull forward. At the same time, disassembly of adhesions at the cell rear is necessary to enable forward progress.
The mechanisms of adhesion assembly and disassembly are still rather poorly understood, but clearly require tight spatial and temporal regulation. The initial clustering of integrins, stimulated by the multivalent nature of the extracellular matrix to which the cell is adhering, is thought to initiate adhesion assembly, but the types of adhesions which form vary greatly depending on the cell type and pliability of the substratum. Rapidly migrating blood cells, such as leukocytes for example, display few integrin clusters and their submicroscopic adhesions facilitate rapid movement. Normally non-migratory or slow moving cells display focal adhesions, large integrin clusters that are tightly adherent and appear to be dependant on Rho-stimulated myosin contractility. Small adhesions, also known as focal complexes, are commonly observed at the leading edge of migrating cells and have been shown to be dependent on Rac and Cdc42. These types of adhesions tend to stabilize lamellipodia and contribute to efficient migration.
Retraction:
The disassembly of adhesions at the rear of the cell and the retraction of the cell's tail complete the cycle of migration and enable cell translocation. Myosin II is crucial for retraction and the development of tension between adhesions at the rear and the retraction machinery. This tension can be sufficient to open stretch-activated calcium channels and lead to the activation of calpain. This protease contributes to adhesion disassembly at the cell rear by cleaving a number of focal adhesion proteins, including integrins, talin, vinculin and FAK. Disruption of PAKa and Rho/Rho kinase signalling, which regulates myosin II, severely impairs retraction in migrating cells. The release of adhesions at the rear of the cell facilitates protrusive activity at the front of the cell, contributes to the overall polarization and provides positive feedback for the continued cycle of migration.
Scratch Assay
Tissue wounds undergo a complex and ordered series of events to repair tissue. These events may include infiltration of inflammatory immune cells as part of the process to remove and destroy necrotic tissue, increased vascularization by angiogenic factors, and increased cell proliferation and extracellular matrix deposition. Wound healing assays have been carried out in tissue culture for many years to estimate the migration and proliferation rates of different cells and culture conditions. These assays generally involve first growing a confluent cell monolayer. A small area is then disrupted and a group of cell destroyed or displaced by scratching a line through the layer. The open gap is then inspected microscopically over time as the cells move in and fill the damaged area. This "healing" can take from several hours to over a day depending on the cell type, conditions, and the extent of the "wounded" region.
The cells must be nearly confluent (~90%) for this to work. For successful wound assays it is critical that everything you do to the cells is done aseptically. Simply put that means you must use agonist, inhibitors, activators or other additives that are filter sterilized. Tips used for scratching must be sterilized. Handling of the cell dishes must be done carefully. Otherwise by the time you get to the most important late time point you will get to take nice pictures of contamination. The changes in morphology at the leading and trailing edge of the cell can tell us quite a bit about the process of cell migration.